Quantum Imaging Laboratory (QIL)
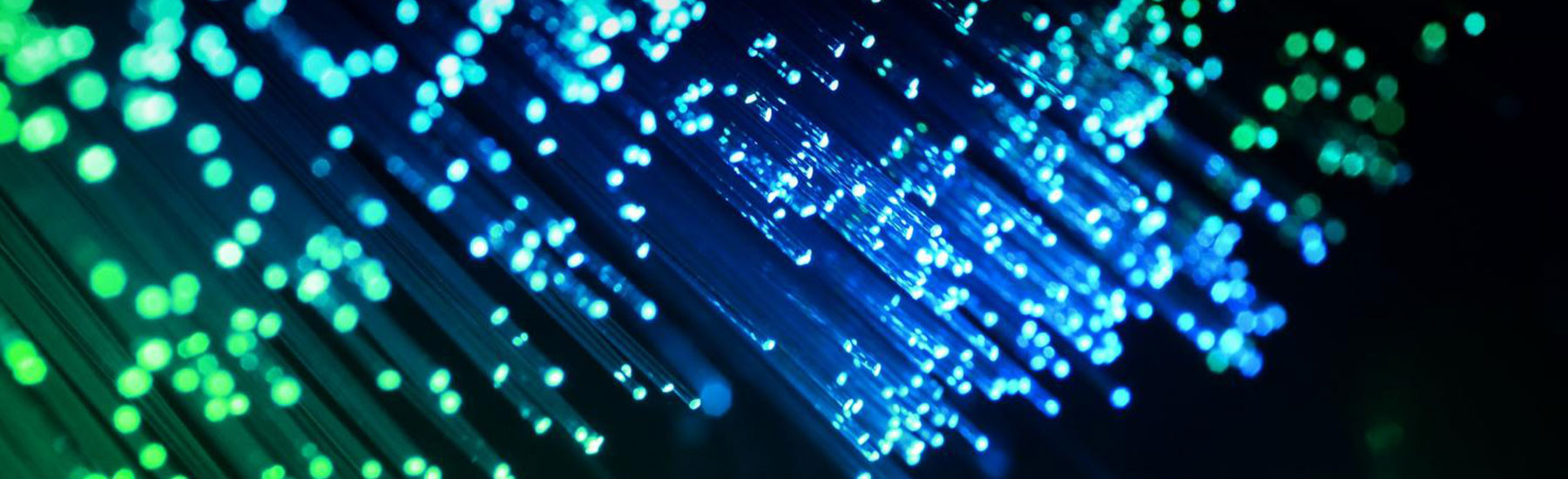
Team leader
-
ORCID ID: 0000-0002-7026-7275
22 55 32 740
Research topics
We apply quantum optical techniques to imaging. We developed a phase imaging technique which is resistant to phase fluctuations. It relies on measuring intensity correlations rather than intensity. Together with the groups of prof. Dan Oron and prof. Yaron Silberberg, we introduced a super-resolution microscopy method based on photon antibunching and we are currently working on its applications in bioimaging.
Members
-
ORCID ID: 0000-0002-7086-4184
22 55 32 746
-
ORCID ID: 0000-0001-9272-8864
22 55 32 747
Quantum correlation fluorescence microscopy
Together with the groups led by Prof. Yaron Silberberg and Prof. Dan Oron we developed two super-resolution fluorescence microscopy techniques based on Image Scanning Microscopy. Both these methods rely on the measurements of photon number correlations. Quantum Image Scanning Microscopy (QISM) exploits the quantum effect of photon antibunching [1] , whereas Super-resolution optical fluctuation image scanning microscopy (SOFISM) relies on fluorescence emitter blinking [2].
Interestingly both the effects occur in commonly used fluorophores, and as a consequence, our methods are relatively straightforward to implement in bioimaging experiments without significantly changing the sample preparation methods.
Moreover, the setup used in both methods is a simple modification of a confocal microscope: a detector (typically a photomultiplier tube) is replaced with an array of detectors. We first demonstrated SOFISM with the help of a fiber bundle connected to multiple SPAD modules and a time tagger. Later in order to bring our new methods closer to real-world applications, we also implemented SOFISM in a straightforward and cost-effective manner by using a SPAD array detector [A Makowski et al., Frontiers in Optics 2020, FW7.E6].
Detection of photons with high spatial and temporal resolution
We developed an intensified camera capable of single-photon detection with high spatial and temporal resolution. We used the camera in two low-light interference experiments and submitted a European patent application on the device itself [8].
Single-photon sensitive cameras are extensively used in modern optics. Intensified cameras provide high spatial resolution and sub-nanosecond gating possibility, but suffer from low duty cycle and high acquisition times when used for observation of random processes. We proposed and experimentally demonstrated a setup in which the number of photons detected within each frame can be controlled. We achieved this by gating the image intensifier adaptively depending on the number of detected events. The use of our system can increase the data acquisition rate and therefore enable photon counting experiments which were not feasible using standard intensified cameras [J Szuniewicz et al, Frontiers in Optics 2020, FW7A.4]. We used our home-built single-photon camera in two experiments:
Quantitative characterization of the spatial structure of single photons [7] is essential for free-space quantum communication and quantum imaging. We introduced an interferometric technique that enables the complete characterization of a two-dimensional probability amplitude of a single photon. Importantly, in contrast to methods that use a reference photon for the phase measurement, our technique relies on a single photon interfering with itself. Our setup comprises a heralded single-photon source with an unknown spatial phase and a modified Mach-Zehnder interferometer with a spatial filter in one of its arms. The spatial filter removes the unknown spatial phase and the filtered beam interferes with the unaltered beam passing through the other arm of the interferometer. We experimentally confirm the feasibility of our technique by reconstructing the spatial phase of heralded single photons using the lowest order interference fringes. This technique can be applied to the characterization of arbitrary pure spatial states of single photons.
Noise resistant phase imaging [J. Szuniewicz et al., Rochester Conference on Coherence and Quantum Optics (CQO-11), 2019, Tu5A.4]: Interferometric methods are essential for making precise measurements and typically require high coherence between the measured and the reference beam. When the phase offset between these beams changes randomly, the interferogram averages out, erasing all the spatial phase information. We proposed and experimentally demonstrated that even when the first-order interference fringes cannot be observed due to the insufficient number of photons within the effective coherence time, the spatial phase of a beam can be measured. Our results show that spatially resolved detection of photon correlations enables holography and interferometry in conditions in which traditional methods fail.
2025
Nanophotonics
Electro-optic frequency shift of single photons from a quantum dot
- S. Kapoor
- A. Rodek
- M. Mikołajczyk
- J. Szuniewicz
- F. Sośnicki
- T. Kazimierczuk
- P. Kossacki
- M. Karpiński
2023
Science Advences 9
Noise-resistant phase imaging with intensity correlation
- J. Szuniewicz
- Kurdziałek /S
- S. Kundu
- Zwoliński/ W.
- Chrapkiewicz/ R.
- Lahiri/ M.
- R. Łapkiewicz
2023
Science Advances 9
Noise-resistant phase imaging with intensity correlation
- J. Szuniewicz
- Kurdziałek /S
- S. Kundu
- Zwoliński/ W.
- Chrapkiewicz/ R.
- Lahiri/ M.
- R. Łapkiewicz
2021
Quantum
Self-referenced hologram of a single photon beam
- W. Szadowiak
- S. Kundu
- J. Szuniewicz
- R. Łapkiewicz
2021
Physical Review A
Characterizing mixed-state entanglement through single-photon interference
- M. Lahiri
- R. Łapkiewicz
- A. Hochrainer
- G.B. Lemos
- A. Zeilinger
2020
Optica
SOFISM: Super-resolution optical fluctuation image scanning microscopy
- A.Sroda
- A. Makowski
- R. Tenne
- U.Rossman
- G.Lubin
- D.Oron
- R. Łapkiewicz
2019
Nature Photonics, 13, 116-122
Super-resolution enhancement by quantum image scanning microscopy
- R. Tenne
- U. Rossman
- B. Rephael
- Y. Israel
- A. Krupiński-Ptaszek
- Y. Silberberg
- D. Oron
2019
Physical Review A, 100, 053839
Nonclassicality of induced coherence without induced emission
- M. Lahiri
- A. Hochrainer
- R. Łapkiewicz
- G. Barreto Lemos
- A. Zeilinger
2017
Phys. Rev. A 95, 033816
Partial polarization by quantum distinguishability
- M. Lahiri
- A. Hochrainer
- R. Łapkiewicz
- G. Barreto
- A. Lemos
- A. Zeilinger
2017
Phys. Rev. A 96, 013822
Twin-photon correlations in single-photon interference
- M. Lahiri
- A. Hochrainer
- R. Łapkiewicz
- G. Barreto
- A. Lemos
- A. Zeilinger
2017
Phys. Rev. A 96,
Twin-photon correlations in single-photon interferenc
- Mayukh Lahiri
- Armin Hochrainer
- R. Łapkiewicz
- Gabriela Barreto Lemos
- Anton Zeilinger
2016
Phys. Rev. Lett. 116, 090405
Automated search for new quantum experiments
- M. Krenn
- M. Malik
- R. Fickler
- R. Łapkiewicz
- A. Zeilinger
2016
Applied Physics B 122, 151
Thermal lens in a liquid sample with focal length controllable by bulk temperature
- Krzysztof Dobek
- Mikołaj Baranowski
- Jerzy Karolczak
- Dariusz Komar
- Kamila Kreczmer
- J. Szuniewicz
2009
Rev. Sci. Instrum. 80, 083101
Collinear interferometer with variable delay for carrier-envelope offset frequency measurement
- M. Pawłowska
- F. Ozimek
- P. Fita
- C. Radzewicz